Bridge Management System Indonesia Pdf
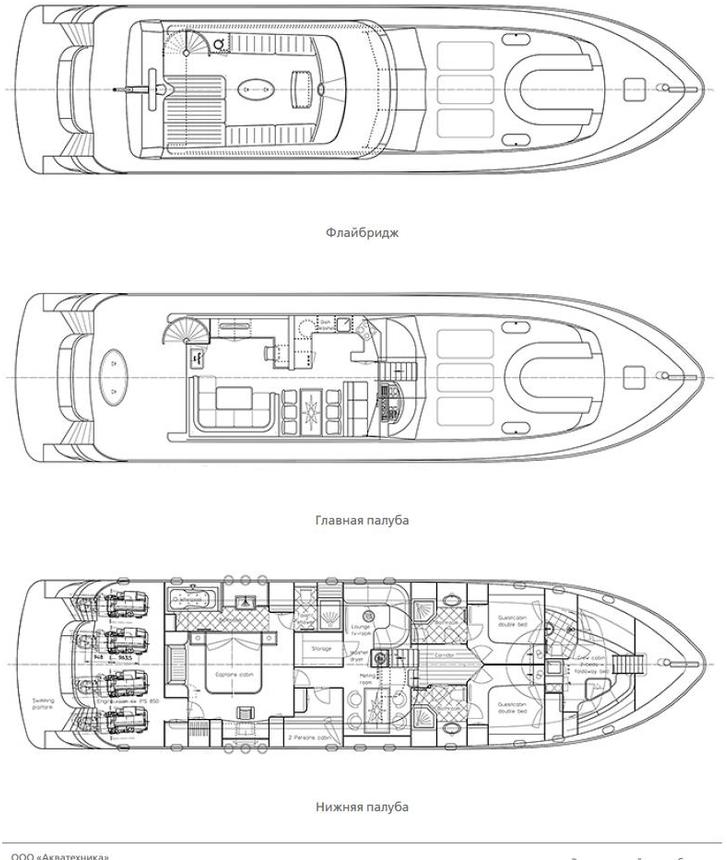
A large number of performance criteria can be derived from a bridge management database, including basic National Bridge Inventory items (4), Pontis analytical products (2), and new measures not currently accounted for in most bridge management systems, such as vulnerability to extreme events.
Mark Hurt, Steven D. Schrock, in, 2016 7.1.2 Definition of Bridge ManagementThe simplest definition for bridge management is that it is the activity of administrating resources to maintain operational bridges. Over several decades, this scale of this activity has increased from managing a few bridges with local labor to making decisions on the state and federal level considering and comparing thousands of bridges at a time. By the 1980s there was significant interest at the state and federal levels in developing efficient and effective bridge management systems (BMS).In contemporary discussions, BMS is often conflated with software. Although software is required to handle the large amounts of data involved in making decisions for inventories of bridges numbering in the thousand, the software is only a tool. Paraphrasing the FHWA report, Bridge Management Systems, a BMS is a system or series of engineering and management functions which, taken together, comprise the actions necessary to administrate resources to maintain an inventory of bridges in operation 5. In practice, typically a number of software applications are utilized to provide the manager with information to further process and make decisions based on engineering judgment.
The flowchart from the 1989 report, remains one of the most complete representations of the complete bridge management process in the literature ( Figure 7.3). The first step is bridge inspection information. This data is at the heart of any BMS.
It is combined with information on construction projects and maintenance activities and associated with specific bridges in a database. In the second activity, information used to conduct an analysis of bridge needs to form a list of prioritized bridge needs and estimated costs to address them. The third activity is to choose projects from among the needs and form a maintenance program. Programs are usually complied on a fiscal year basis, although in times of high needs and low resources programs may be constantly updated to match need with resources as they are available. The fourth activity is to implement the maintenance program and any other actions, such as load posting that are required. The fifth activity is to then evaluate the result of maintenance actions with feedback from all involved parties.
Any changes to policies or practice then are reflected in the next iteration of the process. Flowchart of Bridge Management Activities.
(Adapted from Ref. 5).The activities of the bridge management process are used to direct the bridge preservation cycle of inspection, evaluation, and maintenance. Fl studio flp files download.
The problems of particular bridges are evaluated in the second activity. But prior to selection maintenance projects for inclusion in a program in activity three an evaluation of how the bridge work will fit into system-wide priorities should occur. As bridges are a part of the larger transportation network, a BMS is a part of a larger asset management process.
In evaluating priorities for a highway system there are bridge characteristics that are not related to the structural condition of bridge elements, such as roadway width, which are significant due to their effect on traffic operations. The collapse of the I-35 Bridge in Minneapolis in August 2007 was a focusing event that has changed bridge engineering and management practice in the United States and brought infrastructure into the national dialogue. Technology-based applications such as the following have shown significant cost savings to owners while assuring the efficiency, effectiveness, and reliability of aging infrastructure:.Structural health monitoring.Nondestructive evaluation.Sensing and simulation.Geometry capture and image processingSome state agencies like PennDOT have started utilizing a number of advanced testing and monitoring methods to optimize their inspection, evaluation, and rehabilitation of bridges in the Commonwealth. Examples of these are laser sensors (or so-called LIDAR) to monitor movement of walls and bridges, as well as infrared thermographic technologies to detect delamination in bridge decks.
10.6.1 Recommendations. The following generalized recommendations represent a global approach and are applicable to states in general: 1.Effective maintenance and reducing life cycle costs a.Focus on bridge preservation so that small problems can be corrected before they become significant and costly problems. B.Target the most critical structurally deficient bridges by prioritizing their maintenance, repair, and replacement projects. C.Improve efforts to enforce state and federal truck weight limits to minimize unnecessary damage to bridges due to unpermitted overweight vehicles. D.Continue stricter risk-based weight limitation policies to maintain public safety in light of the aging population of structures. E.Continue the rigorous inspection program that is in place.
Consider national initiatives to put more inspection effort into aging and vulnerable structures, while putting less effort into simpler structures that are in better condition. F.Increase resiliency of the state’s bridge population by gradually replacing or strengthening fracture critical bridges and by replacing aging bridges with structures that are less vulnerable to catastrophic events. 2.Monitoring and inspection a.Investigate the latest technological advancements in structural health monitoring, nondestructive evaluation, sensing, simulation, and other approved innovations to better evaluate the current condition and capacity of the bridge population. B.Supplement conventional bridge design, inspection, and maintenance practice in the case of major, long-span, movable, or complex system bridges. These technologies can also be utilized in identifying of and programming for maintenance needs. 3.Introducing new technology and innovative structural systems a.Update design manuals and construction specifications as new technologies are introduced to the engineering practice. B.Investigate prequalifying contractors based on their ability to handle new technology and by their familiarity with new construction techniques, in addition to the lowest bid amounts.
Mohiuddin Ali Khan Ph.D., M.Phil., DIC, P.E., in, 2015 7.4.1.1 Use of AASHTOware programThe AASHTOware Bridge Rating replaces the former Virtis program. The analytical software allows users to perform bridge load ratings that are indispensable for determining maintenance needs, ensuring public safety, scheduling retrofit or replacement elements, and for assessing overload permits.
Bridge Rating provides highly accurate load rating techniques and calculations.Pontis (now known as AASHTOware Bridge Management) is a bridge management software tool. It is a data application, relying on collected cost data and condition data of bridge elements (beams, piers, railings, etc.).AASHTOware Bridge Management was developed during the early 1990s. AASHTO incorporated the Pontis program into the AASHTOware program and Pontis 4.2 and 4.3 were issued to the licensees in 2003. These data are analyzed to arrive at the optimal long-term preservation and improvement policies for a network of bridges.The software now includes multimedia capability, supports links to photos and drawings, the entering of data in metric or English units, and enhanced security. Pontis 5.1.2 improved the utility of agencies’ bridge inspection data and laid the groundwork for the next generation of AASHTO’s Bridge Management software requirements by ensuring data are in the correct format. It prepares agencies’ inventory data for multiobjective risk assessment, tradeoff analysis, and deterioration modeling tools in Pontis 5.2 (which is currently under development).
Mark Hurt, Steven D. Schrock, in, 2016 1.6 Managing the bridge preservation processPrior to the proliferation of automobiles, bridges on public roads faced the relatively light loads of pedestrians and horse drawn carts. Though there were notable exceptions, most bridge failures, as illustrated by the examples from the early 1900s, did not result in injuries to people. With the need to carry automobile traffic, and the use of steel and reinforced concrete, bridges on public roads became larger and more substantial.
As the first bridges built for such traffic reached several decades of service, the steel and reinforced concrete members suffered from deterioration. The reaction to the failures of these bridges, with the ensuing injuries and loss of life, was the establishment of a national standard requiring regular bridge inspections and evaluations.The activity of managing and scheduling bridge inspections and evaluations, recording and handling bridge data, and making maintenance recommendations became known as bridge management. By 1980, bridge owners had several cycles of bridge inspection under the NBIS, as well as any inspection data retained on their inventory from prior decades. These data allowed owners to make observations on how different bridge types and components – fashioned from different materials – resisted deterioration and challenges from the environment and from traffic. The standardized NBIS format created a nationwide data set for use. In the 1980s North Carolina and a number of states began to explore the use of computerized bridge management systems (BMS) to manage these data 18.
The FHWA sponsored the creation of two BMS systems, BRIDGIT and PONTIS. Development of the PONTIS system has continued and it is currently managed by AASHTO and has been renamed BrM (in reference to bridge management).The development of PONTIS led to changes in bridge inspection practices. To model the progression of deterioration effectively required more refined reporting and recording of inspection findings. Bridge owners who implement PONTIS conduct bridge inspections at the element level.
For example, in lieu of a single numeric value reporting to the condition state of the deck, the area of the deck in each of five defined states of deterioration is recorded. Element level bridge inspection is discussed in Chapter 3.The development of BMS software showed that the data from bridge inspections could be used as the basis for analysis for decisions at the network level. Including deterioration models in the software allowed users to predict future needs for the network of bridges, and to model how different scenarios of maintenance and replacement would affect the condition of the network. Currently, AASHTO defines a bridge management system as, “a system designed to optimize the use of available resources for the inspection, maintenance, rehabilitation, and replacement of bridges” 18.In the past few decades, the scope of bridge management has grown from handling bridge inspection data, to using that data to project the effect of differing funding scenarios on the health of network of bridges. To the primary goal of protecting the safety of the traveling public, has been added the goal of maximizing the effect of maintenance funds on protecting the investment in bridges.As bridge management practices further develop, those involved in the future will need to be concerned with not simply minimizing the cost to maintain the investment in bridges but with calculating the return on the investment in bridges. As illustrated in the discussion of the I-35W Bridge collapse, the failure of bridges can impact the economy of the area served.
The different bridge maintenance funding scenarios modeled in current BMS software result in different levels of bridge functionality across the network. Maintaining bridges in service, but with load restrictions on them results in restrictions on routes which heavy commercial freight may use. Maintaining functionally obsolete bridges in service may result in reduction in traffic capacity on some routes. Justifying the investment in infrastructure such as public roads and bridges is made easier when the benefits are quantified. Pipinato, in, 2016 6.1 Overview on BMSsDue to the rapid growth of automobile and truck usage and the development of massive transportation infrastructures in past decades, there are increasing demands to improve the management methods of bridges, which constitute the most vulnerable elements of the road network. Many agencies responsible for infrastructural networks have recognized the difficulties of the available bridge management approaches, in which decisions are made only on a single project level. As a result, a significant effort has been undertaken in many countries to develop bridge management systems (BMSs) to evaluate the condition of a single bridge in the global network level during its life cycle, and at the same time, to provide efficiency information when allocating resources and establishing management policies in a bridge network.A BMS is a rational and systematic approach to organize all activities finalized to managing network-level bridges ( Hudson et al., 1993).
Decision makers should select optimum solutions from an array of possibilities, and must evaluate and compare alternatives for all bridges in the road network from the viewpoint of life-cycle management in order to avoid similar problems in the near future. Several BMSs have been developed for specific purposes: e.g., Gralund and Puckett (1996) developed a BMS for the rural environment, Markow (1995) developed a BMS for highways, Thoft-Christensen (1995) developed BMS analysis including a reliability approach in particular, and Kitada et al. (2000) developed a detailed BMS for steel bridges. Some studies on evaluation criteria for bridge maintenance that also take into account seismic risk and fatigue evaluation are described in Pipinato (2008a,b).Innovative techniques that include the implementation of new technologies and BMSs have given bridge inspectors and engineers the necessary information to determine an appropriate action. Such a decision is often dependent on a combination of the quantitative information obtained from various measurements, qualitative information obtained from bridge recognition, and general engineering knowledge about the entire bridge system.
In order to allocate funds, a bridge owner needs a BMS that uses historical deterioration trends and predictive relationships. Combining existing management system specifications with bridge specific deterioration models, which consider the system's structural behavior and the aging of the infrastructural network investigated, will improve an infrastructural owner's ability to make bridge specific-decisions and allocate funds for specific and accurate programmed interventions. Probably one of the most significant applications of contemporary BMSs relates to the US.
In 1991, the Intermodal Surface Transportation Efficiency Act (ISTEA) required all states to develop, establish, and implement a BMS by October 1998. The ISTEA requirements, first distributed in 1991, stated that a BMS must be implemented on all state and local bridges. New federal legislation, however, required implementation of BMSs only for bridges on the National Highway System (NHS); therefore, use of BMS inspection for non-NHS bridges was optional ( Sunley, 1995). The principle that BMS used in the US is PONTIS, developed in the early 1990s for the Federal Highway Administration (FHWA), and became an American Association of State Highway and Transportation Officials (AASHTO) practice in 1994. It performs functions such as recording bridge inventory and inspection data, simulating condition and suggesting actions, developing preservation policy, and developing an overall bridge program.
The system allows representation of a bridge as a set of structural elements, with each element reported based on its condition. In 2002, 46 agencies throughout the nation had PONTIS licensing, and each state highway administration (SHA) could customize the system according to its needs ( Robert et al., 2003). BRIDGIT was developed in 1985 by the National Cooperative Highway Research Program (NCHRP) and the National Engineering Technology Cooperation in an attempt to improve bridge management networks.
This system has capabilities similar to the PONTIS system. There have been many research projects throughout the nation on which local agencies have worked with universities to develop their own BMSs.
Other BMSs developed by individual state agencies have good specific functions and qualities, but lack features that can satisfy all the demands of effective bridge management and maintenance procedures on a national scale. Other notable research and development efforts on BMSs took place in Iowa, Washington, Connecticut, Texas, and South Carolina ( Czepiel 1995).
Among recent European experiences that are noteworthy is the TISBO Infrastructure Maintenance Management System, currently being developed by the Netherlands Ministry of Transportation, Public Works, and Water Management. It is a system that integrates inspection registration and maintenance management. Owner agencies in Italy usually manage their network with self-developed codes/procedures regarding BMSs.
The policy of the main Italian agencies is briefly presented in the following:.Rete Ferroviaria Italiana (RFI) is the national agency for the whole Italian railway network, consisting of about 16,000 km. The BMS is based on periodical visual inspections supported by special testing trains. All data are elaborated with specific software developed by the agency with the aim of defining economical and technical convenience of possible maintenance, rehabilitation, and/or strengthening interventions.Autostrade per l’Italia is the most relevant highway agency in Italy; it manages a network of about 3,400 km. The BMS is based on the SAMOA program for surveillance, auscultation, and maintenance of structures.ANAS (1997) is the Italian agency for roads with a national interest, and manages a network of about 26,700 km.
The BMS is based on the national road inventory and in-situ surveys, and is a web-based management application that is developed by the agency and updated regularly.During the last decade, a number of research projects have been financed by the European Commission and some guidelines have been published from these projects that deal with the assessment of existing bridges in Europe; i.e., BRIME (2001), COST345 (2004), SAMARIS (2005), and Sustainable Bridges (2006). All of these guidelines are meant for highway bridges specifically, except for Sustainable Bridges (2006), which is particularly pertains to railway bridges. The purpose of BRIME (2001) was to develop the modules required for a BMS that enables bridges to be maintained at minimum overall cost, taking a number of factors into account, including effect on traffic, life of the repair, and the residual life of the structure. COST345 (2004) investigated the procedures and documentation required to inspect and assess the condition of in-service highway structures, not only bridges. SAMARIS (2005) focused on inventorying the condition of highway structures in European countries, choosing the optimal assessment and strategy selection for rehabilitation through the use of ultrahigh-performance, fiber-reinforced concrete (UHPFRC) and similar technologies. Sustainable Bridges (2006) deals in particular with railway bridges and structural reliability assessment based on in-situ NDT.
Mark Hurt, Steven D. Schrock, in, 2016 6.5 Applying bridge life cycle costing 6.5.1 BLCCA ProgramsThere are three stand-alone programs of note to use for BLCCA. They are: the BLCCA program distributed with NCHRP Report 483 Bridge Life-Cycle Cost Analysis, BridgeLCC from the National Institute of Technology and Standards, and RealCost from the FHWA. Links to all three are available on the FHWA’s website on life cycle cost analysis at: www.fhwa.dot.gov/infrastructure/asstmgmt/lcca.cfm.Each of these programs was originally released prior to 2004. The only program currently being maintained is FHWA’s RealCost. Although this program was originally developed for pavement life cycle cost analysis, it is readily usable for other transportation projects, including those involving bridges. RealCost can calculate agency and user cost and can internally calculate work zone user costs based on FHWA methodology.One feature of note that is shared by all three programs is the ability to assign a probability distribution to the values of cost used in the analysis.
Rather than assign a set value for each cost, the user can assign a range of expected costs and pick a probability distribution (such as uniform, normal, or triangular) based on the user experience with the usual range of bids encountered in contracting. The programs are then capable of running Monte Carlo simulations to determine a range of expected base-year costs.For BLCCA where there are some significant cost that may be quite variable, or when user costs may make a difference in the alternate selected the use of one of these programs may be useful. However, for most BLCCA conducted by bridge owners, spreadsheet calculations will suffice; particularly when maintenance costs collapse into discrete costs at points in time rather than distributed over a period of time. Spreadsheets offer the advantage of requiring the analyst to explicitly know the assumptions made for the analysis.BLCCA is meant to compare alternate scenarios by cost, not to provide values for budgeting. User impacts and vulnerability costs for maintenance work are usually dealt with by policy, that is, traffic accommodations through construction are provided in high AADT areas and emergency routes are designated that are to be operable after extreme event. The typical use of BLCCA for maintenance is to determine the most cost-effective scenario for the agency’s budget.As will be discussed in Chapter 7, modern bridge management programs such as AASHTOWare’s BrM (formally known as Pontis) include the ability to conduct BLCCA for either a single bridge project or to examine inventory-wide maintenance strategies for a bridge owner.
The programs utilize the agency’s bridge inspection data on file with the program and deterioration models for bridge element internal to the program, with cost information provided by the agency, to conduct the analysis. As of yet, this functionality is not widely used by bridge owners. 6.5.2 Typical Applications of BLCCA. The first use may be best illustrated by means of a real life example. During contracted deck repairs to a reinforced concrete deck girder bridge carrying K-177 over Munker’s Creek near Council Grove, Kansas, the deterioration to the existing parapet and deck at the curb line was found to be significant enough so as to require removal of the parapet with the bridge railing ( Figure 6.10).
The reinforcing steel had corroded to the point where the parapet was liable to separate from bridge with a vehicular impact. Almost all the reinforcing steel between the deck and the parapet would have to be replaced. The most economical repair alternative would be to remove and replace the entire length of bridge rail and parapet on each side of the bridge. This added work would cost more than the bridge work in the original contract bid. Considering the age of the structure, it was not apparent as to whether replacement of the rail would be a more efficient use of funds rather than installing temporary barrier and replacing the bridge as soon as prudently possible. Paul Kulseth, Bridge Management Engineer for the KDOT conducted a BLCCA comparing two scenarios with a review period ending in 2070: 1.Proceeding with a cost overrun of the current substantial maintenance project and replacing the existing bridge rail and parapet with new permanent bridge railing.
A subsequent deck and joint repair project was assumed at a 15 year interval with replacement of the bridge assumed at the end of its project 75 year life. Another deck and joint repair project was assumed to occur when the new bridge was 15 years old. 2.Ceasing work on the current substantial maintenance project. The work would be renegotiated to removal of the existing rail and parapet and placing temporary concrete safety barrier in place at temporary bridge rail. The bridge would be replaced in 5 years, with subsequent deck repairs (no joints were presumed in the new design) at 15 year intervals. BLCCA for Munker’s Creek Bridge.With the spreadsheet, it was possible to examine the effect of changing assumptions in the timing of maintenance and replacement actions.
Doing so, it was calculated that replacing the rail with permanent railing would be the most economically efficient solution for any scenario in which the bridge would not be replaced for 13 years.As an example of the second common use of BLCCA, Afshin Hatami and George Morcous completed a study of scenarios for deck overlays, expansion joint replacements, and deck widening versus deck replacement projects for the NDOR to determine the most cost efficient strategies 19. The analysis was conducted using RealCost, an analysis period of 60 years and construction costs from NDOR. Deterioration rates of bridge elements were taken from the deterioration rate report for Nebraska bridges by the same authors 2 years earlier 15.For deck overlay, strategies involving three different types of overlay were investigated: silica fume overlays, epoxy polymer overlays, and polyester overlays.
For silica fume overlays, three scenarios were compared: allow a deck to deteriorate until it reached NBI condition state 4 and then redeck, applying a silica fume overlay at condition state 6, and applying a silica fume overlay at condition state 5. It was determined that the least net present value was achieved with application at NBI condition state 6.Neither the epoxy polymer overlay nor the polyester overlay are meant to be placed on a severely deteriorate deck. The authors assumed that each would be placed when a deck reached 15 years in service. Given the shorter service life of these two overlay types, additional applications of each would have to be made during the 60 analysis period. To match the net present value provided by applying a silica fume overlay, it was determined that an epoxy polymer overlay would require a service life of at least 14 years and a polyester overlay would require a service life of 22 years. Yadin David, Zhou Dan, in, 2016 Overview of Clinical Engineering and Healthcare Technology Management MarketFrom innovation to retirement, safe and effective technology’s life cycle requires collaboration and knowledge sharing between many stake holders.
The stake holders include the inventors, manufacturers, regulators, distributors, installers, service personnel and maintainers, integrators, and users, to name a few. Regardless of the stake holder field of practice, competencies and skill structure all share in the commitment to “do no harm” and in the potential for conflicts of interest ( Smith and Sfekas, 2013). Healthcare delivery in general and patients in particular expect that, like other professionals, clinical engineers be competent and ethical in their practice. Thus, when it comes to healthcare technology, clinical engineers serve as guardians of patient safety and quality care outcomes.Life cycle healthcare technology management must integrate the following stages: Technology compliance, technology planning, technology assessment, acquisitioning support, installation services, commissioning, training, scheduled and on-demand service support, risk management, upgrades, replacement/retirement.Healthcare technology has made improvements both in quality patient outcomes and in improving patient experience ( Johnson, 2012). Healthcare technology management bridges the gap between theory and innovation and point-of-care practices where the service of clinical engineers is focused ( Shemer et al., 2005). Clinical engineering program services aim at optimally managing the technology and to create sustainable environment of safe and efficient application of the technology in support of care services. The technology can vary from mostly mechanical device like patient scales to intensive intelligence-embedded equipment like robotic surgical system and various imaging modalities that range from ultrasound to Magnetic Resonance Imaging (MRI) and Positron Emission Tomography (PET) systems.The market scope of healthcare technology management and servicing programs is mostly divided between Original Equipment Manufacturer (OEM) service organization, Third party or Independent service organization (ISO), and In-house service program (Clinical Engineering).
Healthcare technology management and servicing of the technology can be provided by one of these service providers or in the alternatives as a combination of more than one of the providers. Variations in needs, resources, and location contribute to difference in program’s terms such as scope of services, cost of service, response time, and quality. In selecting the provider, or providers, there should be systematic evaluation prior to initiating any implementation of these programs. Regardless of the program source, all of them depend on the competencies and skills of clinical engineering professionals to repeatedly and professionally delivering service excellence. The global growing in volume of healthcare services, and thus technology that is deployed at these care delivery institutions, creates global demand for educated and well-trained clinical engineering professionals. Bureau of Labor Statistics predicts that highest percent growth (30% increase) of future jobs will be in the medical equipment servicing and ranked this job opportunity as the best during this decade ( U.S. Bureau of Labor Statistics, 2015).
The many phases of healthcare technology management program demand adequate engineering and management education that includes training also in communication, writing, and project management. Clinical engineers around the world who possess these skills are advantageously positioned for pursuing satisfying and critically important career.The Government of China embarked on improving affordable and expanding access to healthcare services for its population through the National Healthcare Reform Plan. Additional USD123 billion has been invested with the goal of funding hospitals, make services in rural and urban areas equally available, and establish national medical devices system ( Zhou and Ying, 2013).
The anticipated growth of clinical engineering profession under such an environment can only be diminished by lack of clinical engineering expertise.China, by 2020, expects to surpass the United States, will have the largest economy in the world, and by 2025, China’s nominal GDP will hit USD38.563 trillion. This economy will be characterized by high consumption spending, strong currency rates, and favorable trade ties ( Sinclair, 2009). China’s healthcare sector is also developing at an astonishing rate. This remarkable growth is largely attributable to the country’s increasing government spending, underpinned by robust economic growth, which has led to improved healthcare access and infrastructure, as well as the ongoing expansion of public insurance coverage and infrastructure for less developed parts of the country ( Han, 2012).
Beyond the growth in government spending, patients’ ability to afford better medical care has increased ( McKinsey & Company, 2014). Driving this investment is the country’s rapidly increasing middle class and its aging population, which are both increasing the demand for healthcare. It behooves on global clinical engineers in the academia, government, hospitals, and industry areas that if they desire to reach full appreciation for such enormous reform plan impact, then they also be cognizant of influences in China, such as cultural ones, have on professional practices. Boulent Imam, in, 2019 6.4 Climate Change Versus Asset Stock Data UncertaintiesMany infrastructure operators worldwide manage large numbers of assets. The number of rail bridges in the European Union is approximately 217,000 ( Bell, 2004), while the total number of highway bridges in the United States is over 600,000 ( ASCE, 2013). Infrastructure operators often manage bridge stocks with limited budgets through prioritisation techniques based on risk assessments, using network- and asset-specific data.
However, when managing a large asset base, maintaining reliable and up-to-date data records can be a challenging task. The challenge is exacerbated when the infrastructure is old and robust data records may have been lost or nonexistent; for example, most of the 28,000 bridges on the British railway network were built in the 19th century ( Bell, 2004). Thus, asset managers often need to make decisions on the basis of incomplete and uncertain information, prompting the need for a robust risk management framework ( Piyatrapoomi et al., 2004 ). Numerous additional uncertainties from various sources affect the decision-making process in bridge management. Notably, climate change is notoriously uncertain and its effects on infrastructure are still not well understood by engineers.This section explores uncertainty associated with data used in bridge scour risk assessments at a bridge stock level. This uncertainty is explored in the context of a changing climate, to explore the propagation of climate change uncertainty through the scour risk assessment and its interaction with other sources of uncertainty.
To understand the relative importance of different parameters on the scour risk of bridges, sensitivity analyses have been carried using a number of United Kingdom railway bridges as case studies and considering the United Kingdom (Network Rail) railway scour assessment code. Scour assessments in the United Kingdom take place at two stages; stage 1 is a preliminary assessment, which all bridges over water are subjected to. Any bridge highlighted as being at risk is assessed at stage 2, which includes a detailed hydrological and hydraulic assessment and a more robust data collection process. Local scour is estimated through an empirical model, similar to the one presented in Section 6.3. Scour risk is estimated in terms of a final priority rating (FPR) which is a dimensionless value that is used to compare the relative risks at different structures, so that they can be prioritised with respect to scour vulnerability and criticality.
A more detailed description of the procedure and the models used for the sensitivity analysis can be found in Dikanski et al. 6.16 shows a summary of the results from the sensitivity analysis for the different parameters that have been investigated for the case study bridges analysed. The diagram shows the overall change of risk score FPR resulting from variations across the entire studied parameter ranges, which have been identified by comparing data between stage 1 and stage 2 assessments, as explained in Dikanski et al. The most influential parameters are shown to be FD, pier or abutment width and floodplain width. Angle of attack is also significant for pier scour, but not for abutments.
Sensitivity analysis for piers ( top) and abutments ( bottom).It was also found that, in most cases, the errors in parameters would lead to an underprediction of actual risk, meaning that exposure to scour risk is likely to be higher than current assessments suggest. Errors in floodplain width records are shown to have the largest effect on the estimation of risk both for piers and abutments.Overall, parameters that are often assumed to be deterministic in the literature were found to be subject to large and variable uncertainties due to the data used for assessments. In the case of unknown FD, the high resulting uncertainty affecting scour risk estimates is a well-known issue; the results presented above re-emphasise that it is one of the largest sources of uncertainty.
Furthermore, some additional parameters, for example, abutment width and floodplain width, are also found to have a major influence on the estimation of scour risk. In practice, this reduces the confidence in scour assessments, which determine investment priorities for scour interventions. By understanding the sensitivities of a scour risk model to its inputs, infrastructure operators can target their efforts in reducing the uncertainty in the most significant parameters, thereby increasing confidence in scour assessments.
Thus, scour intervention schemes can truly target the highest priority sites and the value of infrastructure investments can be maximised, while risks are effectively reduced. As a result of research presented in this chapter, a review of bridge data used for scour assessments globally is recommended. This would be an essential first step towards improving bridge resilience both in the context of current and future climate.Climate change is associated with significant uncertainty, which is often quoted as a major barrier to increasing climate resilience.
However, results show that resulting changes in river flows have a relatively low impact on the estimation of scour risk, as compared to other parameters. This is due to several reasons, including the use of high initial assessment flows (200-year return period), exclusion of flow considerations from contraction scour estimation and exclusion of cumulative effects of scour. In practice, the increase of flood frequency and severity expected to be brought about by climate change is likely to increase scour risk, but this is not being detected by the selected case study model, as shown by a range of previous studies ( Khelifa et al., 2013; Wright et al., 2012; Nemry and Demirel, 2012; Dikanski et al., 2016; HR Wallingford, 2014). In order to begin to understand the true impacts of climate change on bridge scour risk, asset managers need to reduce epistemic uncertainties, associated with bridge data, which currently dwarf the effects of climate change uncertainty on scour risk assessments. This would be a valuable climate change adaptation measure and an essential first step towards increasing bridge resilience in the context of current and future climate.Although for bridges with unknown foundations, bridge data uncertainties have been found to be even more influential, compared to climate change uncertainties, this may not be the case with bridges with known foundations or better defined asset data. For the latter, the climate change uncertainties may become relatively more significant; an extension of the current work can provide insight in these relative differences.
The last five years have seen the art of bridge management develop into a mature subject. Bridge owners and engineers recognise the importance of implementing fully operational bridge management strategies to ensure that all road and rail bridges remain functional for as long as possible.Bridge structures form a major part of the vast financial investment in infrastructure and consequently their careful management involving structural appraisal, repair and strengthening is of paramount importance.